- Plants and animals provide a home within themselves to an invisible community of microbes known as the microbiome. But these natural microbial communities are being degraded and altered by human-caused biodiversity loss, pollution, land-use change and climate change.
- On the macro level, habitat loss and diminished environmental microbe diversity, particularly in urban environments, is altering the gut microbiomes of humans and wild animals. Studies have linked microbiome changes to higher risk of chronic and autoimmune diseases.
- Coral bleaching is an extreme example of climate stress-induced microbiome dysfunction: During heat waves, beneficial microbes go rogue and must be expelled, leaving the coral vulnerable to starvation. Microbiome resilience is key to determining corals’ ability to acclimate to changing ocean conditions.
- There are solutions to these problems: Inoculating coral with beneficial microbes can reduce bleaching, while the restoring natural green spaces, especially in socioeconomically deprived urban areas, could encourage “microbiome rewilding” and improve human and natural community health.
Scientists, policymakers and the public are becoming increasingly aware that biodiversity loss, climate change, deforestation and pollution are causing profound disruptions to Earth’s ecosystems.
But every animal and plant that makes up these ecosystems could also be considered a diverse ecosystem in their own right, with each providing a home to a little-explored, invisible community of microbes — living both on, and inside every individual — known as the microbiome.
Today, as microbiome research extends beyond biomedical applications into conservation and ecology, scientists are finding unseen wonders, but also something disturbing: This microscopic world is gravely threatened by human activities.
Scientists are beginning to map a micro-biodiversity crisis unfolding in the guts of humans and animals that only biodiversity-enhancing solutions can solve. They’re also finding that these microorganisms are having an outsized influence on us as well, and that macro and micro worlds depend on each other for health.
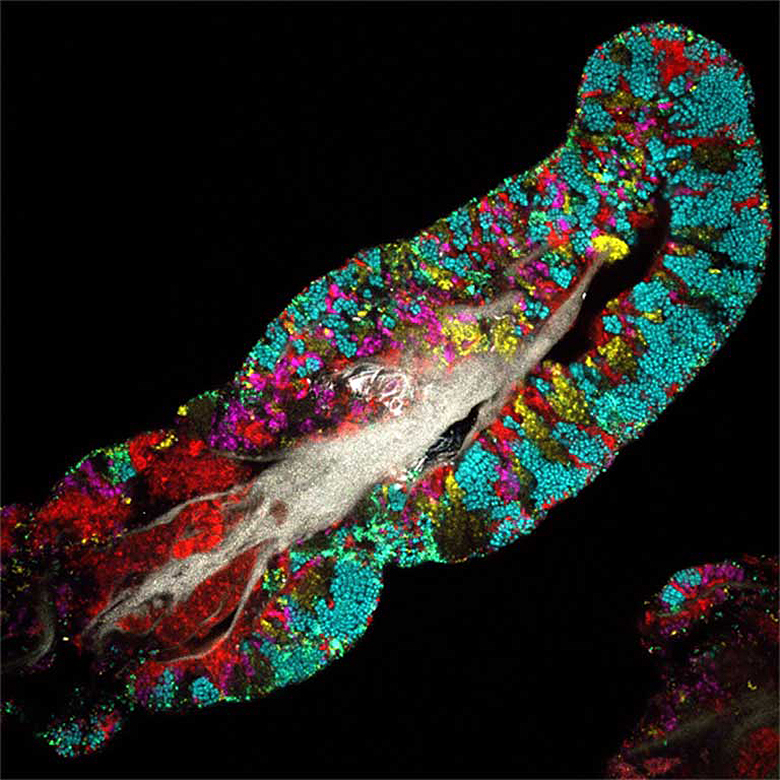
The invisible ecosystem inside you
The term microbiome refers to the diverse community of bacteria, single-celled archaea, fungi and viruses that live on, or inside, other living organisms. These microbial hitchhikers can make up a significant proportion of their host’s body weight: The human microbiome, for example, weighs about 2 kilograms (4.4 pounds).
We support a varied microbiome — on our skin, in our airways, and along the length of the digestive tract — but the gut microbiome is the largest, best-studied, and believed to be the most important.
People often think of gut microbes as harmful; E. coli, for example is a common cause of food poisoning. But many gut microbes are not only beneficial but vital for breaking down food nutrients and producing useful compounds absorbed into the bloodstream. Gut microbes play complex roles in digestion, immunity and even cognition — relationships that research is just beginning to shine a light on.
One relationship that is well established is the interaction between the gut microbiome and health. In lab animals and humans, dysfunction of the gut microbiome has been linked to digestive diseases (such as ulcerative colitis, inflammatory bowel disease and Type 2 diabetes), allergies and autoimmune diseases (such as asthma, hay fever and rheumatoid arthritis), along with contributing to neurological and mental health problems (including Alzheimer’s disease, depression and anxiety).
Understanding the diverse species in the gut microbiome, their interactions, and their functional roles in providing immunity and preventing disease has become an important goal for researchers and clinicians. But that knowledge base is also turning out to be a moving target because microbiomes are always changing and evolving, especially as these natural systems are impacted by human influences.
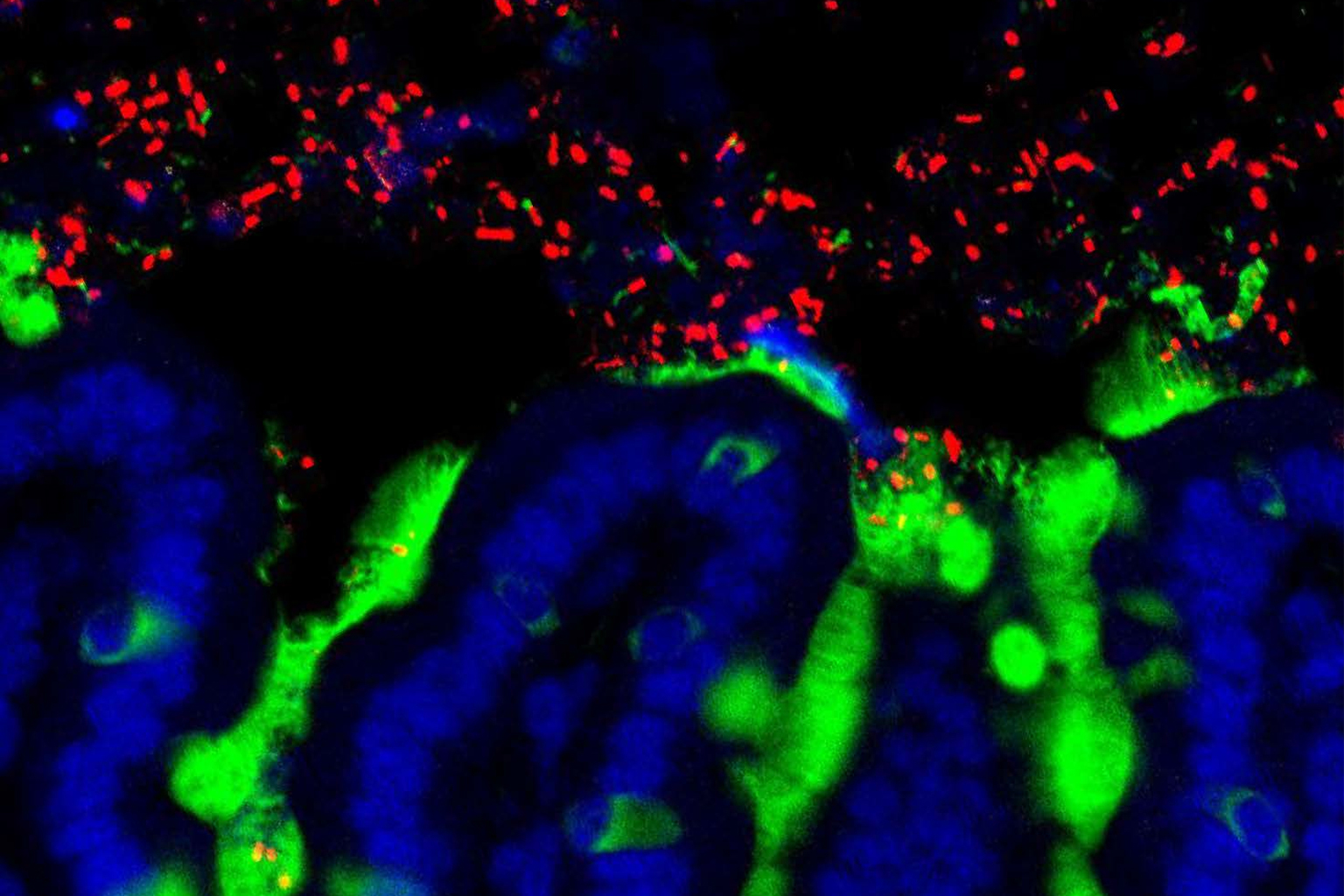
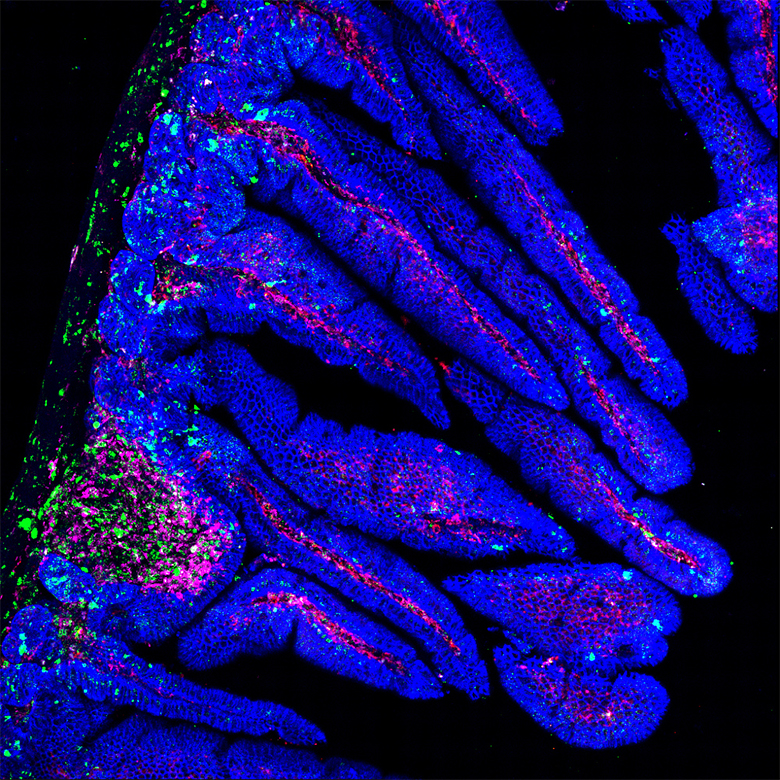
Healthy ecosystems seed diverse microbiomes
What factors determine which microbial species make a home in our guts? It’s complicated.
Researchers do know we aren’t born with them: We acquire our microbial ecosystem starting at birth and develop it throughout life via exposure to microbes in our diet and surrounding environment. In fact, the environmental influence on our microbiome is so profound that identical twins share only 37% of the same gut microbes, despite their shared genetics.
So it follows logically that the health and diversity of the microbes thriving in the external environment have a big impact on the diversity and health of the gut flora cultivated within us. Martin Breed, a restoration ecologist at Flinders University in Adelaide, South Australia, says there is “strong evidence that there is an effect of [local] biodiversity on people’s gut microbiome.”
“We’re breathing in the microbes that are in the environment,” microbes that may then take up residence in our bodies, he explains.
But not all environmental microbiomes are created equal: More biodiverse natural ecosystems harbor more beneficial microbes and offer a richer pool from which we can assemble a healthy microbiome.
Given this intimate link between environmental biodiversity and the composition of the microbiome, it’s perhaps not surprising that habitat loss and land-use change are altering the gut microbiomes not only of people, but of wild animals. One study, for example, found that black howler monkeys (Alouatta pigra) inhabiting evergreen rainforest in Mexico harbored more beneficial bacteria compared to individuals living in fragmented habitats.
In fact, urbanization is known to be driving gut microbiome change in both humans and animals: A study of fecal microbiota from people, anole lizards, and coyotes in Puerto Rico found that urban humans and animals share many of the same gut microbe species, distinct from the gut microbiota of their rural counterparts.
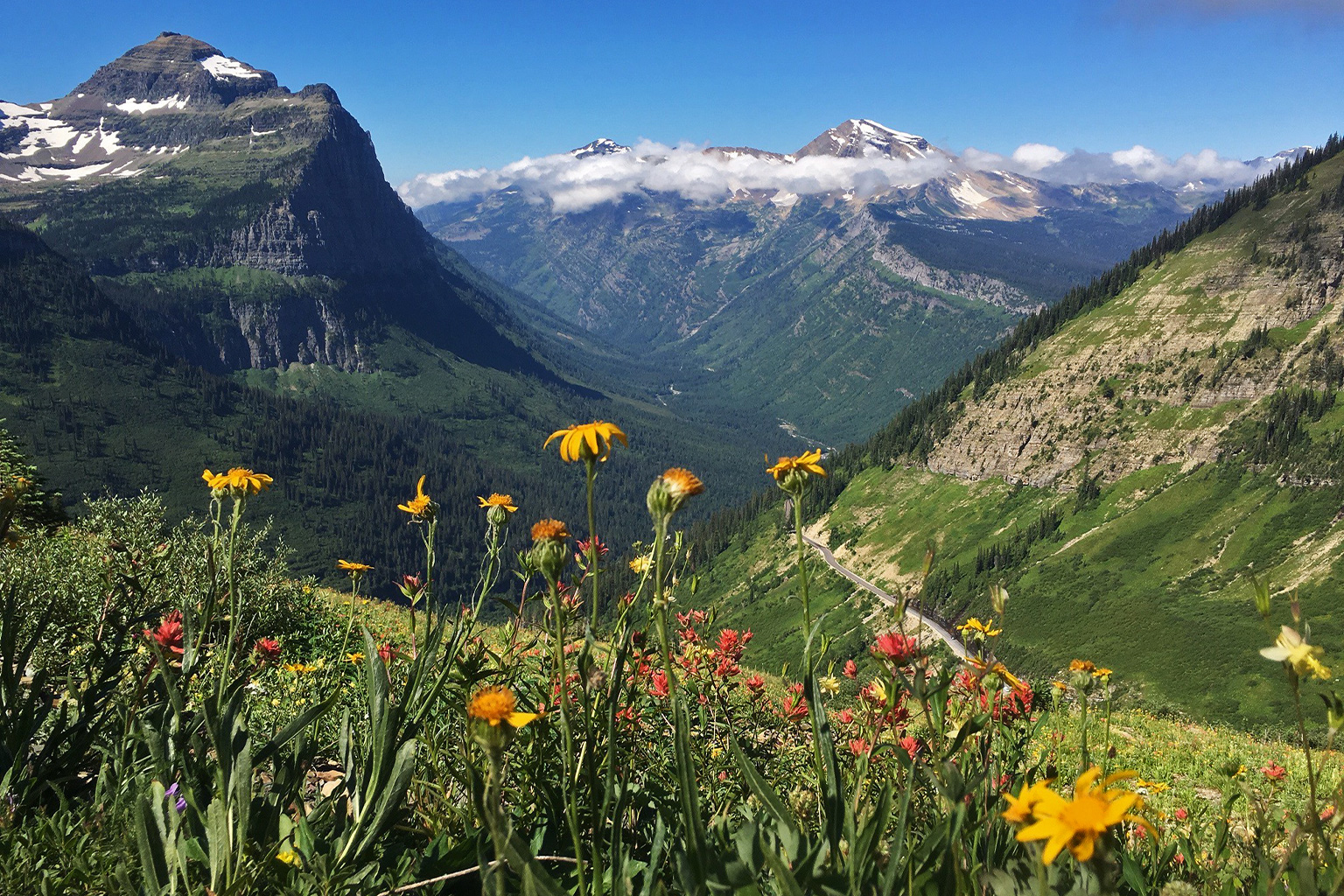
Urban lifestyles reduce environmental microbe exposure
“One of the things we get from the natural environment and from soil is [bacterial] spores,” says Graham Rook, professor of clinical microbiology at University College London. These organisms “drive the regulatory arm of the immune system that stops it from attacking things it should not attack, such as our own tissues or harmless allergens.”
Numerous studies have linked reduced exposure to environmental microbes in cities to an increased risk of the chronic and autoimmune diseases that are proliferating in urban environments, particularly in industrialized nations.
When scientists first began to notice the link between environmental microbe exposure and human health and immune function, they hypothesized that over-cleanliness could be to blame for the explosion of allergies and autoimmune diseases in Western industrialized nations. The “hygiene hypothesis” became popular in the 1980s and ’90s, but decades of research has shown that the problem is not the lack of pathogen exposure in the home, but a lack of exposure to diverse beneficial microbes in the environment — the result of increasing urbanization and chemical pollution, the introduction of processed foods, and many other microbe-dampening facets of modern life.
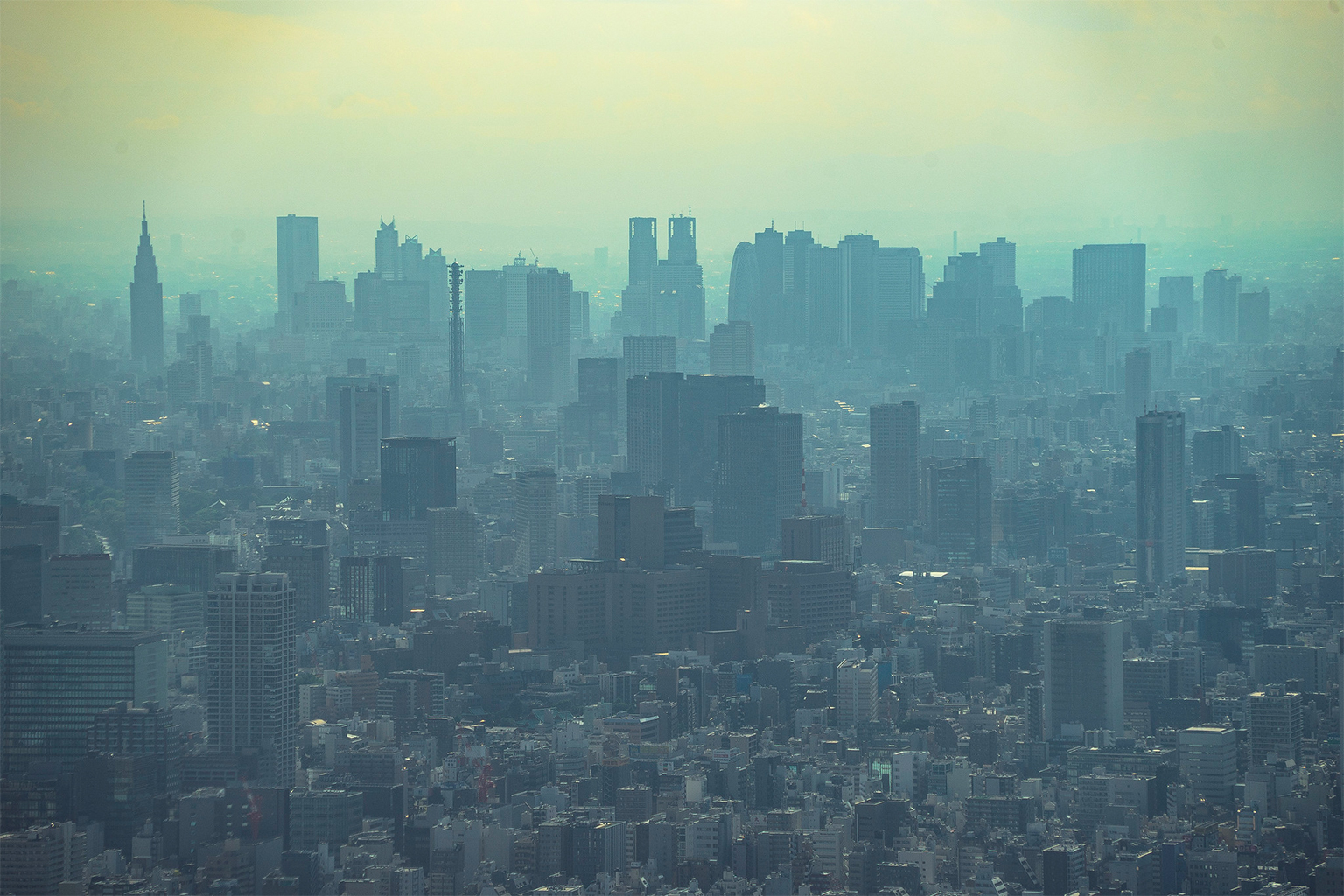
Not only do urban environments limit our exposure to the beneficial microbiota of natural ecosystems, but we also “bulldoze our metaphorical rainforest with antibiotics,” creating a “clean slate [in our guts] which provides all sorts of opportunities for the wrong taxa to get their foot in the door,” explains Breed.
Once established, these harmful microbes may enjoy a competitive advantage over beneficial colonists, making it difficult to reverse gut microbiome damage once it’s done.
“We do need to encounter the microbiota of [our] mother, and the microbiota of the natural environment, particularly in early life, when the immune system and the gut microbiota are being established,” says Rook. Humans co-evolved with these microbes over millennia and “they are essential for setting up many aspects of the physiology of the infant.” The influence of environmental microbes on our microbiome continues throughout our lives.
That’s why good hygiene targeted toward key settings — in the kitchen, bathroom, and during surgery, for example — is fundamental to public health. But important too is exposure to biodiverse natural ecosystems. But deforestation and other land-use changes are devastating biodiversity globally and turning rich natural ecosystems into agricultural monocultures and sprawling metropolitan districts. That makes replenishing our own natural microbiome far more challenging.

Transgressed planetary boundaries driving microbiome change
Biodiversity loss and land-use change represent two of the nine planetary boundaries — the operating system processes that have kept conditions on Earth stable and habitable for human civilizations over the past 12,000 years.
But our transgressions of these and other planetary boundaries (including climate change, ocean acidification, atmospheric aerosol pollution, and pollution by novel chemical entities) are also disrupting the relationship between plants, animals, people and their microbiota.
An example: Polar bears (Ursus maritimus) in East Greenland, where year-round sea ice allows these predators to feed on their natural diet of seals, have a gut microbiome that’s distinct from that of polar bears in the Beaufort Sea off Alaska, where melting sea ice has forced populations to spend more time foraging on land. Researchers found that the East Greenland bears had higher levels of Bacilli and Coriobacteriia, bacterial groups thought to be important in maintaining gut health. As humans change the biosphere around us, we are likewise changing our gut flora and fauna.
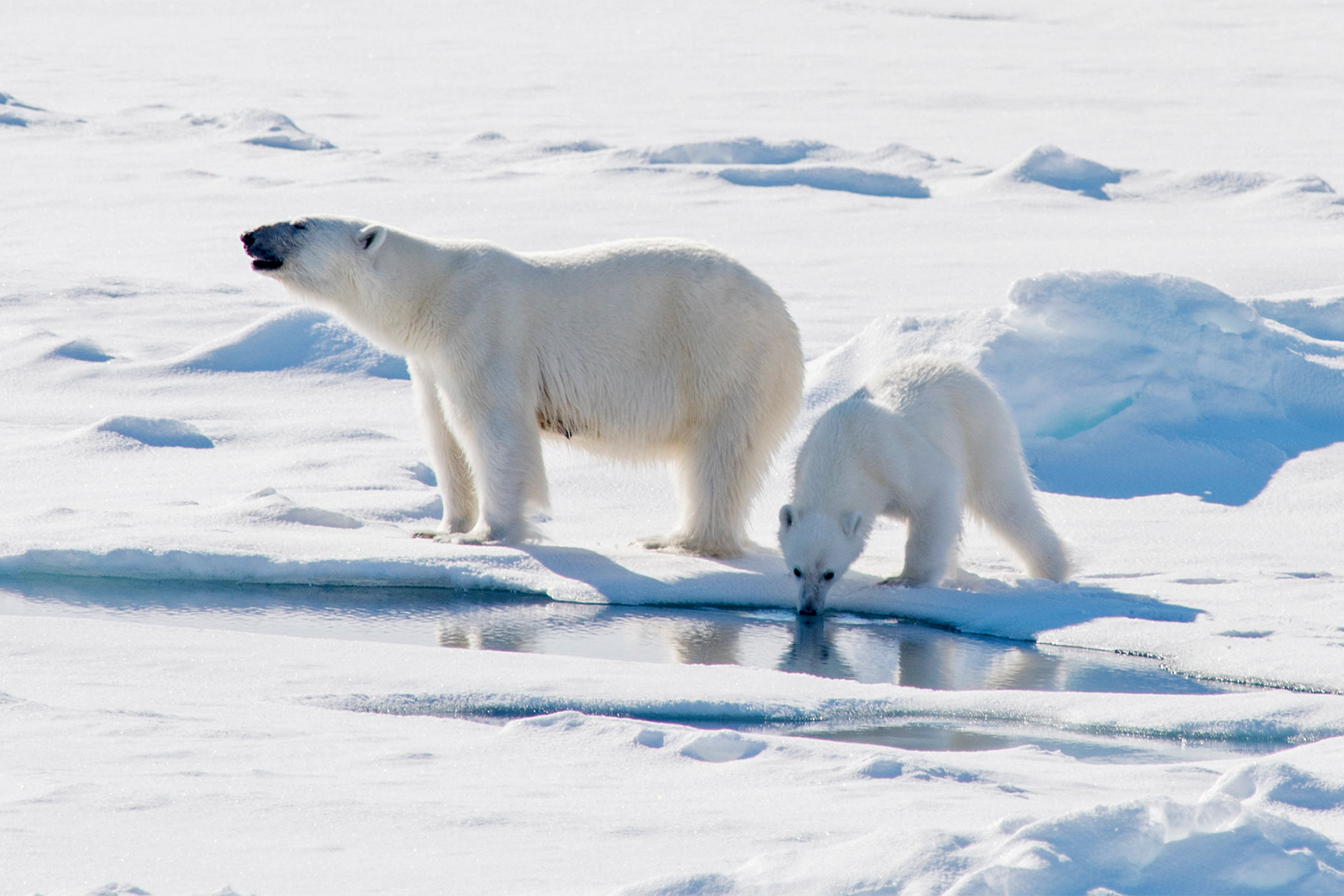
Coral reefs: Good guys gone rogue
While we know that environmental degradation can reshape and degrade the microbiome, the converse is also true: Changes in microbiome composition can have serious knock-on effects on biogeochemical cycling and worsen greenhouse gas emissions, helping destabilize the planet.
And it isn’t just species composition that determines the function or dysfunction of the microbiome: Benign or even beneficial microbes can go rogue in response to stress, ceasing their helpful role or even becoming parasitic to their host. For example, stress-induced competition between host and symbiont is responsible for coral-bleaching events that have become an increasingly familiar sight in the tropics as heat waves become more frequent and severe with climate change.
Coral reefs are formed via a “partnership between the coral host itself, which is the animal, and the algal symbiont, [along with] a vast array of bacterial communities associated with corals,” as well as fungi and viruses, explains marine microbial ecologist David Bourne of James Cook University in Queensland, Australia. The corals’ algal symbionts, members of the Symbiodiniaceae family, provide the majority of the corals’ food.
However, rising water temperatures “break down the symbiosis between the coral and its Symbiodiniaceae,” Bourne explains. During heat waves, the algae become overactive and parasitic, forcing the coral to expel them in self-defense. The coral can survive a short time without the algae, and if water temperatures drop again, the coral can pick up new microbes from the environment.
But “if it’s for a long time then they can actually starve to death,” says Bourne.
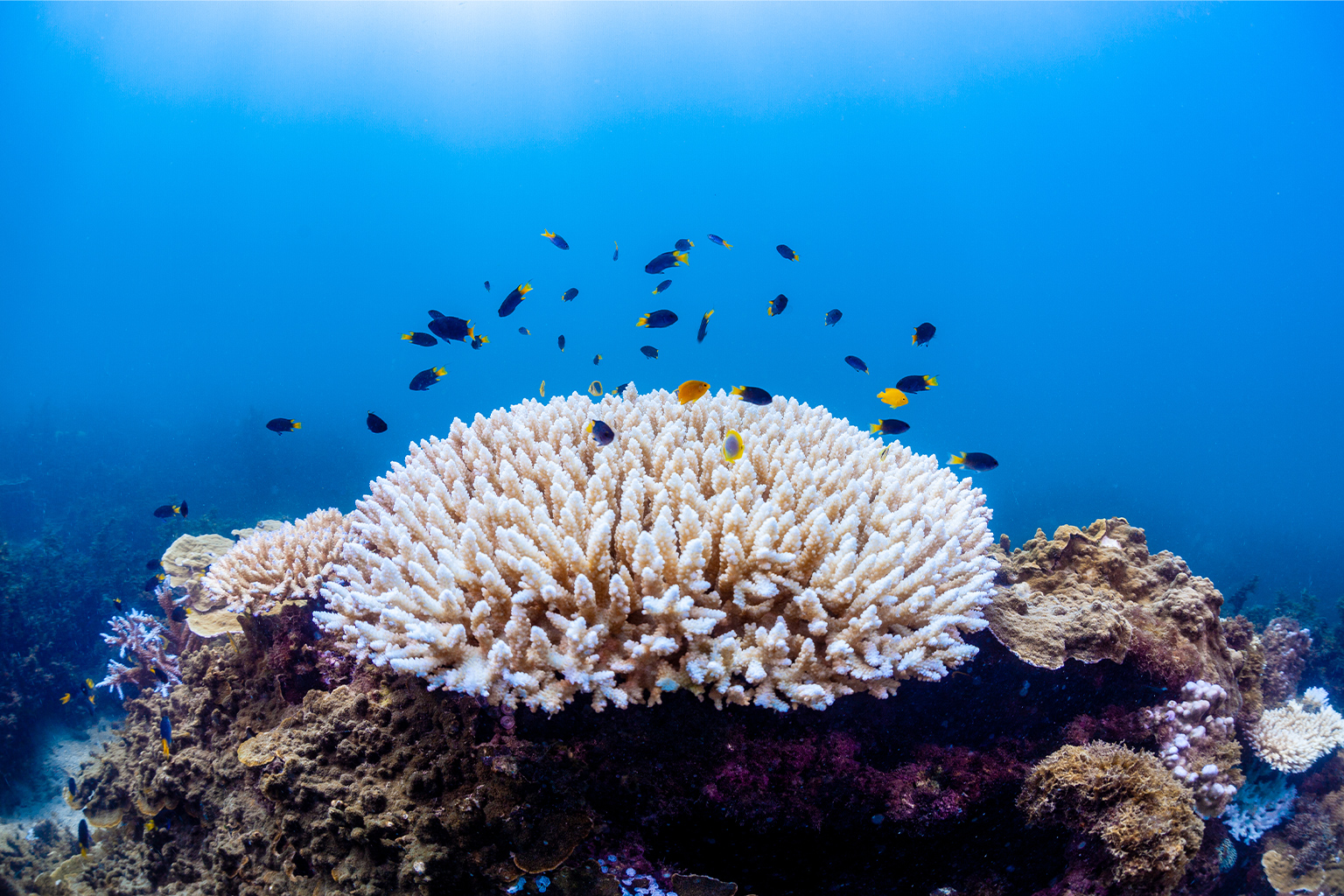
Approaching ecosystem tipping points
Coral-bleaching events continue becoming more frequent as ocean temperatures rise, and researchers warn that reefs may reach a tipping point beyond which they can’t recover.
“As we get to 2 degrees [Celsius, or 3.7° Fahrenheit] warming, that’s where coral reefs really suffer,” Bourne cautions. Increases above that level will trigger widespread bleaching events, without giving sufficient time to recover, resulting in massive die-off of corals, he says: “The system changes.”
This widespread microbiome failure could sound the death knell for many of the world’s coral reefs — one of Earth’s most biodiverse ecosystems which provide vital nurseries to commercial fisheries — and could bring starvation to parts of the world. This coral algae example demonstrates graphically just how far the destructive ripples of a catastrophic microbiome failure can spread.
But there’s hope: Some corals are more resilient to bleaching than others, and research shows that their microbiome is an important factor in determining the ability of corals, and other marine organisms, to acclimate to changing ocean conditions. The acclimatization of some corals to elevated ocean temperatures has already been linked to their heat-tolerant symbionts, and some species can shift their microbiome in favor of more resilient microbial strains as temperatures rise.
Similarly, the resilience of sponges (another key species group in the reef ecosystem) against ocean acidification has been linked to the pH tolerance of their microbial symbionts. Also, thanks to the functions of their microbiome, sponges are integral to ocean nutrient cycling and carbon sequestration. As a result, they are a key component in Earth’s operating system, helping to keep our transgressions of both the climate change and biogeochemical flows (nitrogen pollution) planetary boundaries in check.
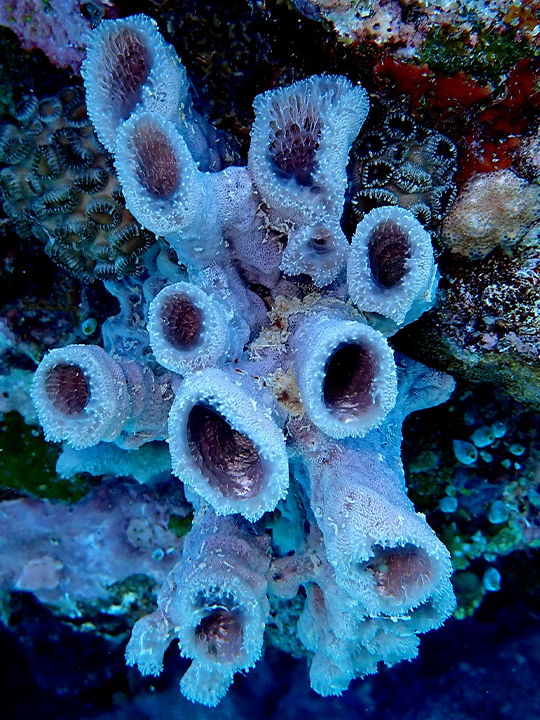
“Sponges, with their immense filtering capacity — thousands of liters per day — form a critical link between benthic [seafloor] and pelagic [open sea] environments, and are involved in a number of important biogeochemical processes,” says Heidi Luter, a research scientist at the Australian Institute of Marine Science in Queensland.
Microbial symbionts allow sponges to assimilate carbon trapped in decaying organic matter, providing an important source of nutrition for the sponge and — later when dead sponge cells are shed — for the entire reef ecosystem. Many sponges are also host to archaea, single-celled organisms “involved in the nitrogen cycle and carbon fixation,” says Luter. These archaeal symbionts may also “increase the resistance of sponges to anthropogenic stressors.”
Research into sponges demonstrates that the relationship between microbiome composition and planetary boundary stability is a two-way street. While the environment is known to shape microbiomes in positive and negative ways, likewise a healthy microbiome can play a vital role in regulatory functions of ecosystems that keep the whole planetary system stable.
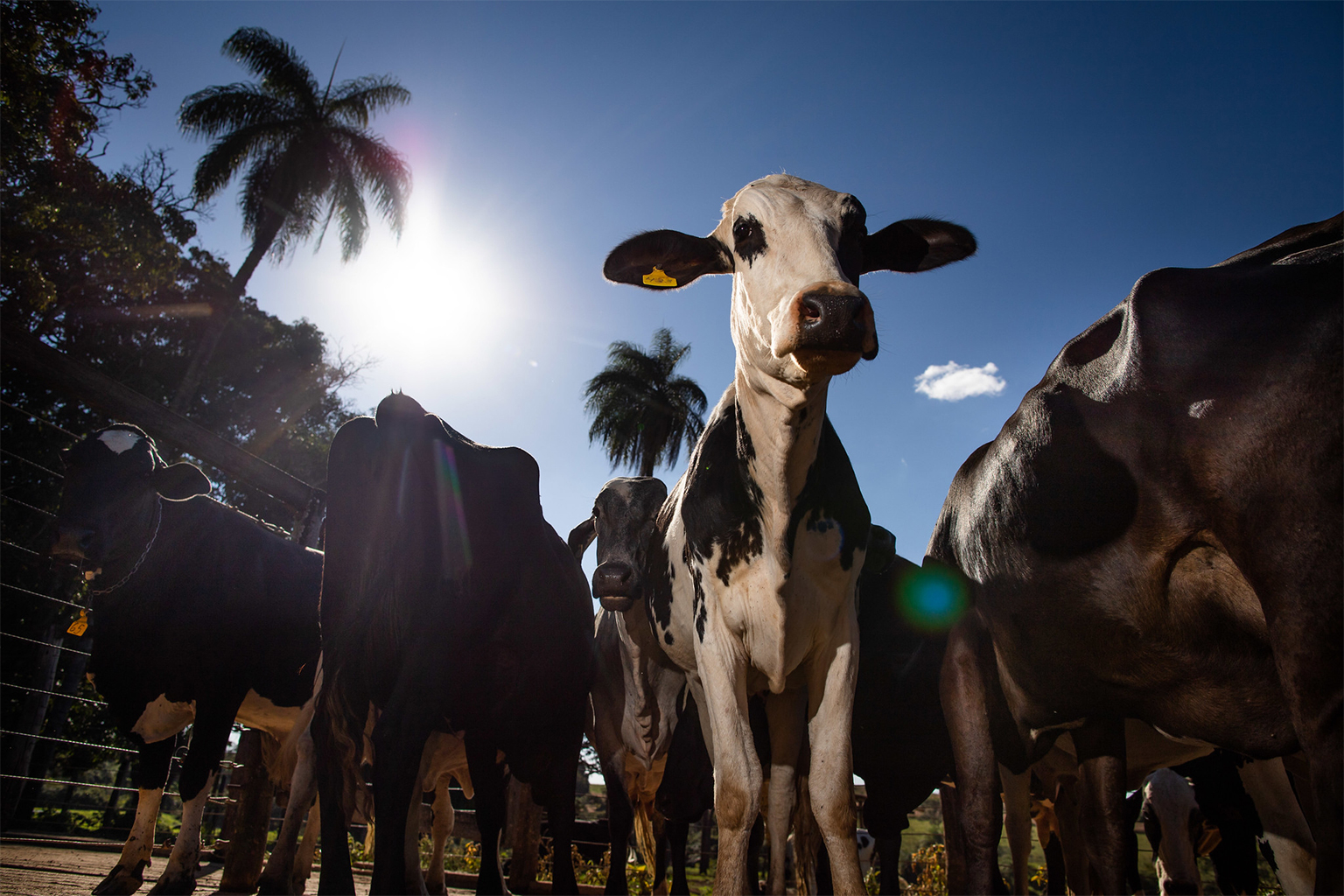
Microbiome is central to livestock carbon emissions
An example from agriculture further illustrates just how powerful an influence a particular microbiome can have on Earth’s environment. Cattle are a major greenhouse gas emitter, and their rearing for the meat and dairy industry is helping destabilize the climate planetary boundary. The cause of this imbalance is their diet and its impact on their microbiome.
Cattle and sheep are ruminants, meaning they can digest plant matter in a specialized stomach called the rumen. “The complex microbial community that exists in the rumen is critically important for the digestion of feed into high-quality nutritious meat and milk products for human consumption,” explains molecular biologist Sinead Waters, a professor at the Teagasc Animal and Bioscience Research Department in Ireland.
However, “as part of that digestion process, a byproduct called methane gas is produced,” she adds.
Methane is a potent greenhouse gas with a 20-year climate warming potential — 84-86 times that of CO2. Its emission in the burps of ruminant livestock, mainly cattle, is responsible for 40% of global greenhouse gas emissions from agriculture. This methane production is also inefficient, wasting up to 12% of the animals’ energy intake.
So cutting rumen methane emissions has become a key goal of agricultural scientists hoping to both reduce the climate footprint of livestock, while also increasing bovine growth and milk production. There are two routes to these objectives: selective breeding or dietary changes to promote a less methanogenic microbiome.
“There has been a recent proliferation of research on strategies to mitigate methane emissions from agriculture,” Waters states. “One of the most promising approaches in the shorter term is the development of anti-methanogenic feed additives,” which could significantly reduce gut methane emissions.
Waters explains that “diet plays a major role in altering the rumen microbiome, and less methane [is] generally produced when cattle are fed high-energy expensive concentrate diets [than] high-forage dietary regimes.” However, Waters’ research team has found that adding white clover to the grass alters the rumen microbiome, reducing the abundance of methane-generating bacteria. Like coral reef sponges, cattle and their microbiome, when nurtured properly, have the potential to significantly curb climate change.
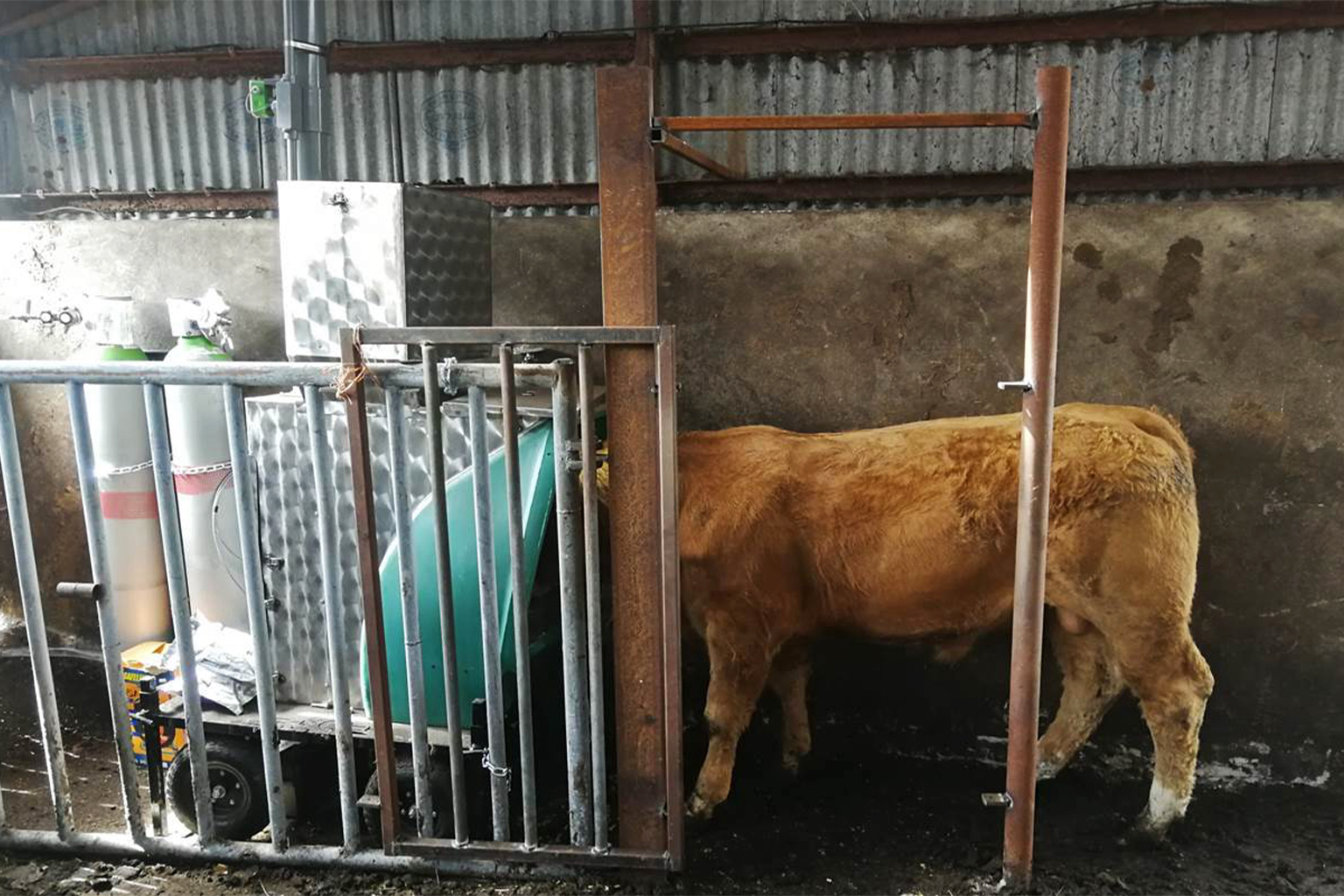
Microbial probiotics and beyond
Our growing understanding of the many perils confronting our gut microbiome continues to lead to new therapeutics and preventative interventions that aim to restore and maintain animal microbiome health.
Some microbiome-based therapeutics are already available for humans, ranging from dietary probiotics of varying efficacy, to fecal bacteriotherapy, a technique that transplants gut microbes from a healthy donor to treat recurrent bacterial infections. While such high-tech microbial transplant options aren’t yet available for ailing corals or wildlife in fragmented habitats, researchers are hard at work developing alternative remedies to support healthy and resilient microbiomes in the wild.
One example: The inoculation of coral with a cocktail of beneficial microbes has been shown to reduce the likelihood of temperature-induced coral bleaching in aquarium experiments. Biologists hope that by engineering a more resilient coral microbiome, they can help mitigate the stresses of climate change, ocean acidification and pollution on reefs, improving resilience and survival rates.
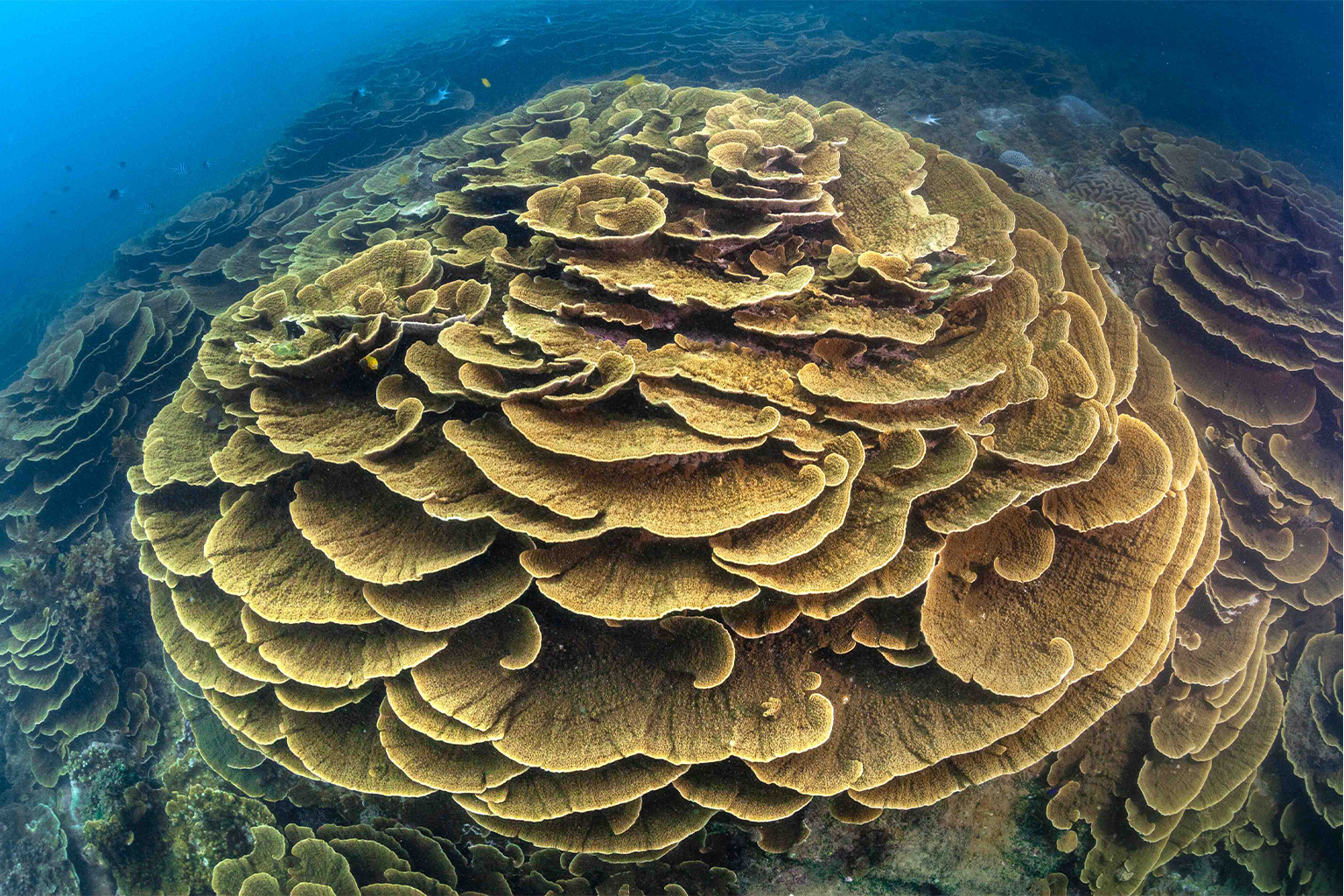
“The real critical research question is to work out how adding something like a probiotic improves coral health and fitness,” says Bourne. By understanding which microbes benefit coral resilience and why, “you could actually package a food or a diet that the coral can then uptake.”
This emphasizes a major challenge slowing the development of microbiome-based solutions: It’s extremely difficult to study any microbiome and tease apart mechanistic relationships between species composition and function in order to target potential treatments. That’s partly because many microbes, being adapted to life in an oxygen-free gut environment, can’t be studied at all in isolation.
“Many of the rumen microbes are unculturable in the laboratory,” explains Waters, meaning that their biology remains largely unknown.
Nevertheless, “Finding the mechanism of action [for bovine] feed additives is critical in proving their effectiveness [in reducing methane emissions],” she says. “Feed additives such as seaweeds and [commercially produced] Bovaer are proposed to work by inhibiting the effects of the enzymes involved in methane production,” allowing for additive tailoring to maximize the inhibitory effect.

Microbiome rewilding meets restoration ecology
Rather than treat the symptoms of poor human microbiome health with pills or probiotics, restoration ecologists have proposed a holistic preventive remedy, known as “microbiome rewilding.”
This technique, inspired by restorative conservation practices, rehabilitates natural spaces at the macro level to cultivate a diverse pool of environmental microbes so they can seed healthier microbiomes in humans and wildlife.
Take worn-out farmland, for example: “Classical restoration ecology treatments, like revegetation, native plant replanting, taking care of invasive species and pests” could be employed to restore the soil microbiome in degraded ecosystems, says Breed. “The co-benefits would be not just for the environment, but for people via the microbiome.”
For many city dwellers, a few natural urban green spaces, such as New York’s Central Park, provide their only regular exposure to semi-wild ecosystems. So enhancing biodiversity in these urban places, and increasing their locations across cities, could be beneficial in enhancing environmental microbe diversity, thereby improving the human microbiome and ultimately reducing the incidence of chronic immune diseases.
Researchers in Finland found evidence this can work by bringing forest soils and vegetation into day care center playgrounds, which altered the gut microbial community of children and lowered inflammatory markers in their blood after just 28 days. Breed commends the study, describing it as “the best available evidence of direct exposure to biodiverse microbiomes from the environment changing the gut [microbiome] of … children and shaping their immune system.”
The potential positive impacts of increased exposure to natural ecosystems may even extend to directly improving our mental well-being. Breed’s research has shown, for example, that in mice, exposure to tiny quantities of biodiverse soils can have a positive impact on the gut microbiome as well as behavior.
Exposure to “high-biodiversity soil significantly shifted the gut microbiome of the mice,” Breed emphasizes. Afterward, the female mice “showed a reduction in their anxiety-like behavior,” which “we traced [to] a butyrate-producing bacterium from high-biodiversity soil [that traveled] into the gut of these mice via the air.”
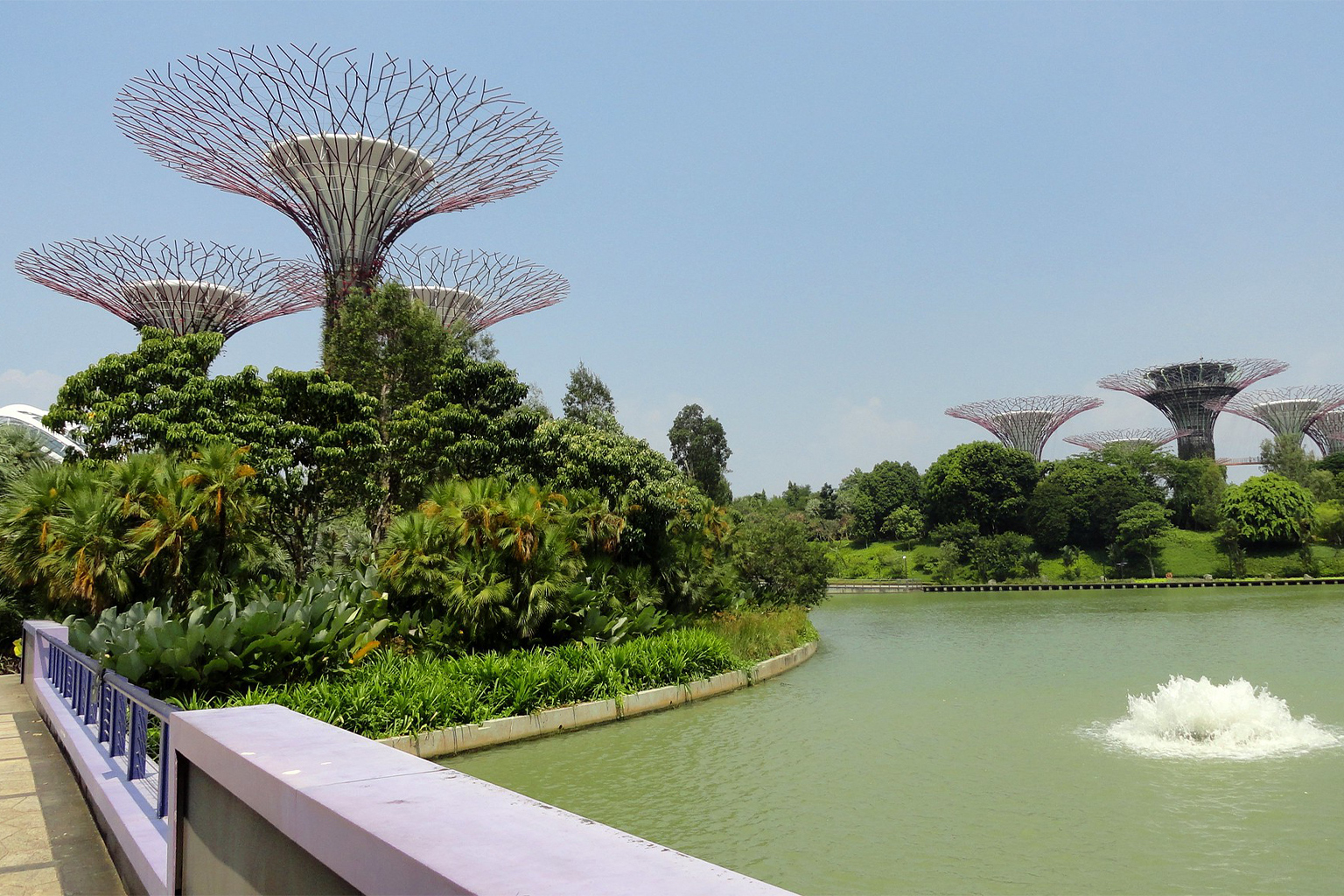
Can we have a positive gut reaction to nature?
Pioneering studies like this one with mice show that microbiome influence extends far beyond the gut and all the way to the mind. It adds to decades of research into the deep connection between the digestive system and the brain, known as the gut-brain axis. This biochemical signaling pathway between the gastrointestinal tract and central nervous system influences our moods and behaviors, our cravings and repulsions.
Breed thinks our microbiome may be influencing us even beyond that. Studies have shown that people who spend more time interacting with nature during childhood are more likely to show nature-loving or biophilic traits as adults. The “lovebug hypothesis” suggests that this positive feedback loop might be directly driven by symbiotic gut microbes that we pick up from the outdoor environment.
This outdoor interaction may not only be beneficial to humans, but also good for gut microorganisms, and in the self-interest of both: After all, these microbes “are reliant on us to complete their life cycle,” Breed notes, and he speculates wryly as to whether they may be “manipulating us to like going back out into nature.”
People who grow up with more access to, and better connections with, nature “do all of the things that you would expect them to do if the microbes were hijacking them and getting them to go outside to complete their life cycle,” Breed laughed.
If such interactions between the micro and the macro are common, then urban microbiome rewilding projects, especially in socioeconomically deprived areas, could help to inspire a love and appreciation of nature in a new generation of city children and address some of the socioeconomic disparities in health and well-being at the same time.
Microbiome research represents a bold new frontier for science, with each new study adding to the validity behind the old adage “We are what we eat,” while establishing new truths such as: “We are what we breathe,” and “We are what we experience,” and maybe much more.
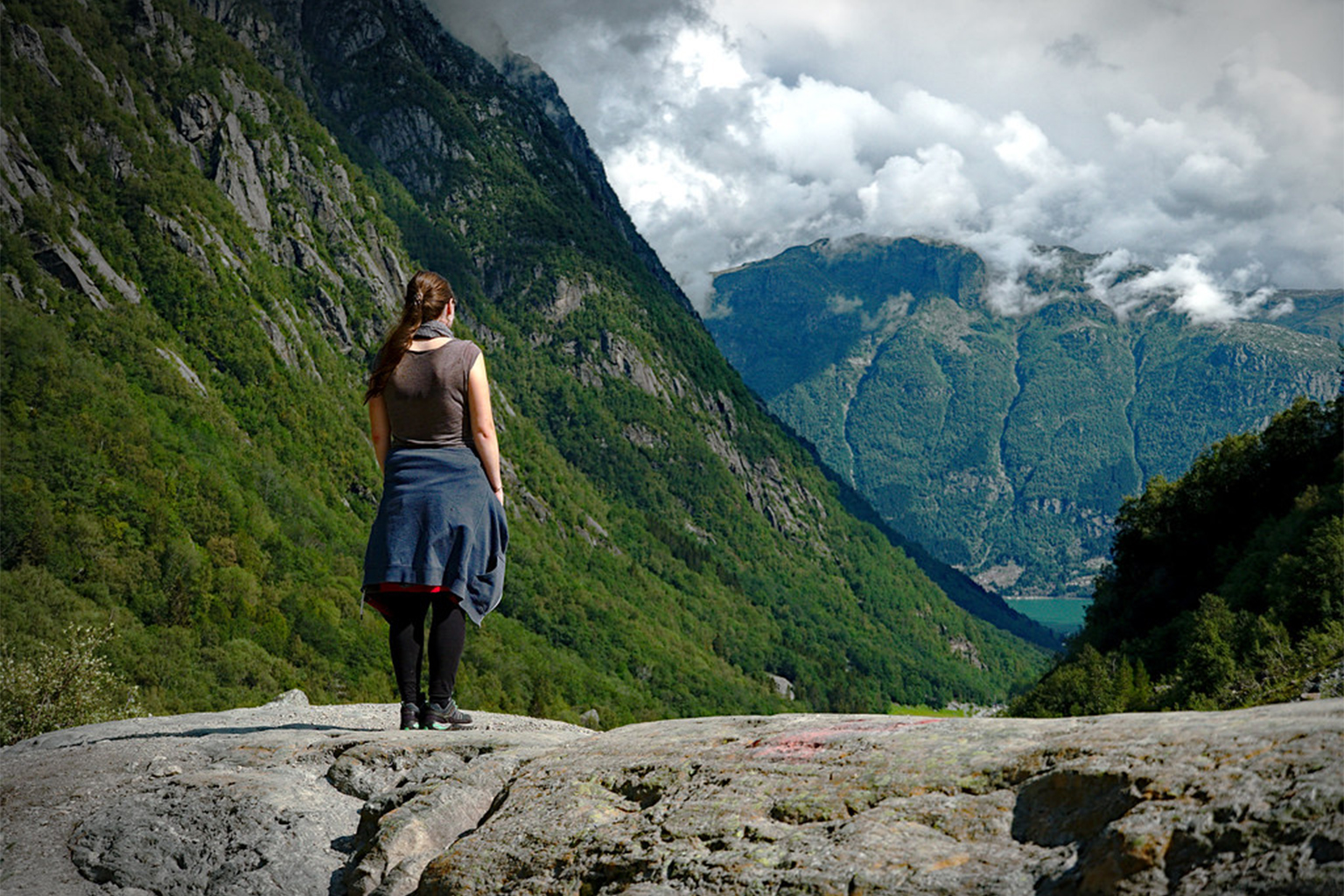
Banner image: In lab animals and humans, dysfunction of the gut microbiome has been linked to digestive diseases (such as ulcerative colitis, inflammatory bowel disease and type II diabetes), allergies and autoimmune diseases (such as asthma, hay fever, and rheumatoid arthritis), along with contributing to neurological and mental health problems (including Alzheimer’s disease, depression and anxiety). Image courtesy of Pacific Northwest National Laboratory via Flickr (CC BY-NC-SA 2.0).
Citations:
Amato, K. R., Yeoman, C. J., Kent, A., Righini, N., Carbonero, F., Estrada, A., … Leigh, S. R. (2013). Habitat degradation impacts black howler monkey (Alouatta pigra) gastrointestinal microbiomes. The ISME Journal, 7(7), 1344-1353. doi:10.1038/ismej.2013.16
Dillard, B. A., Chung, A. K., Gunderson, A. R., Campbell-Staton, S. C., & Moeller, A. H. (2022). Humanization of wildlife gut microbiota in urban environments. bioRxiv. doi:10.1101/2022.01.05.475040
Haahtela, T. (2019). A biodiversity hypothesis. Allergy, 74(8), 1445-1456. doi:10.1111/all.13763
Strachan, D. P. (2000). Family size, infection and atopy: The first decade of the “hygiene hypothesis”. Thorax, 55(90001), S2-S10. doi:10.1136/thorax.55.suppl_1.s2
Rook, G. A. W., Lowry, C. A., & Raison, C. L. (2015). Hygiene and other early childhood influences on the subsequent function of the immune system. Brain Research, 1617, 47-62. doi:10.1016/j.brainres.2014.04.004
Rook, G. A. W., & Bloomfield, S. F. (2021). Microbial exposures that establish immunoregulation are compatible with targeted hygiene. Journal of Allergy and Clinical Immunology, 148(1), 33-39. doi:10.1016/j.jaci.2021.05.008
Franz, M., Whyte, L., Atwood, T. C., Laidre, K. L., Roy, D., Watson, S. E., … McKinney, M. A. (2022). Distinct gut microbiomes in two polar bear subpopulations inhabiting different sea ice ecoregions. Scientific Reports, 12(1), 522. doi:10.1038/s41598-021-04340-2
Grottoli, A. G., Warner, M. E., Levas, S. J., Aschaffenburg, M. D., Schoepf, V., McGinley, M., … Matsui, Y. (2014). The cumulative impact of annual coral bleaching can turn some coral species winners into losers. Global Change Biology, 20(12), 3823-3833. doi:10.1111/gcb.12658
Berkelmans, R., & Van Oppen, M. J. H. (2006). The role of zooxanthellae in the thermal tolerance of corals: a ‘nugget of hope’ for coral reefs in an era of climate change. Proceedings of the Royal Society B: Biological Sciences, 273(1599), 2305-2312. doi:10.1098/rspb.2006.3567
Reisinger, A., Clark, H., Cowie, A. L., Emmet-Booth, J., Gonzalez Fischer, C., Herrero, M., … Leahy, S. (2021). How necessary and feasible are reductions of methane emissions from livestock to support stringent temperature goals? Philosophical Transactions of the Royal Society A: Mathematical, Physical and Engineering Sciences, 379(2210), 20200452. doi:10.1098/rsta.2020.0452
Smith, P. E., Enriquez-Hidalgo, D., Hennessy, D., McCabe, M. S., Kenny, D. A., Kelly, A. K., & Waters, S. M. (2020). Sward type alters the relative abundance of members of the rumen microbial ecosystem in dairy cows. Scientific Reports, 10(1), 9317. doi:10.1038/s41598-020-66028-3
Santoro, E. P., Borges, R. M., Espinoza, J. L., Freire, M., Messias, C. S. M. A., Villela, H. D. M., … Peixoto, R. S. (2021). Coral microbiome manipulation elicits metabolic and genetic restructuring to mitigate heat stress and evade mortality. Science Advances, 7(33). doi:10.1126/sciadv.abg3088
Roslund, M. I., Puhakka, R., Grönroos, M., Nurminen, N., Oikarinen, S., Gazali, A. M., … Sinkkonen, A. (2020). Biodiversity intervention enhances immune regulation and health-associated commensal microbiota among daycare children. Science Advances, 6(42), eaba2578. doi:10.1126/sciadv.aba2578
Liddicoat, C., Sydnor, H., Cando-Dumancela, C., Dresken, R., Liu, J., Gellie, N. J. C., … Breed, M. F. (2020). Naturally-diverse airborne environmental microbial exposures modulate the gut microbiome and may provide anxiolytic benefits in mice. Science of The Total Environment, 701, 134684. doi:10.1016/j.scitotenv.2019.134684
Robinson, J. M., & Breed, M. F. (2020). The Lovebug Effect: Is the human biophilic drive influenced by interactions between the host, the environment, and the microbiome? Science of The Total Environment, 720, 137626. doi:10.1016/j.scitotenv.2020.137626
FEEDBACK: Use this form to send a message to the author of this post. If you want to post a public comment, you can do that at the bottom of the page.
Unseen crisis: Threatened gut microbiome also offers hope for world
Source: Trends News
0 Comments